The severe acute respiratory coronavirus 2 (SARS-CoV-2) of the Coronaviridae family is the causative agent of the coronavirus disease 2019 (COVID-19) global pandemic that has caused more than 6.5 million deaths from over 600 million recorded infections.
SARS-CoV-2 is closely related to SARS-CoV and the Middle East respiratory syndrome coronavirus (MERS-CoV), both of which led to epidemic outbreaks in 2002-2003 and 2013-2014, respectively, and are associated with significant morbidity. Human infections have also been reported from other coronaviruses, such as OC43, 229E, NL63, and HKU1, most of which manifest as the common seasonal cold.
Study: New insights into human immune memory from SARS‐CoV‐2 infection and vaccination. Image Credit: Kateryna Kon / Shutterstock.com
Background
SARS-CoV-2 enters the host by binding to angiotensin-converting enzyme 2 (ACE2) receptors on the surface of host cells through its receptor-binding domain (RBD) within the viral spike protein. ACE2 receptors are present in the cells of the respiratory tract, gastrointestinal tract, heart, and kidneys.
Most people who contract SARS-CoV-2 experience mild respiratory symptoms. However, patients with pre-existing comorbidities, such as chronic obstructive respiratory disorder (COPD), obesity, asthma, and immunocompromised individuals, are at a greater risk of severe COVID-19. For example, those with increased ACE2 expression or impaired immune function exhibit higher viral loads, infectivity, and poor viral control.
Scientists have studied human immune responses against SARS-CoV-2 infection. Recently developed technologies can detect, quantify, and phenotype immune memory cells. The emergence of SARS-CoV-2 variants of concern (VOCs) has emphasized the need for biomarkers capable of quantifying the protection conferred by COVID-19 vaccines based on the initial Wuhan strain.
A recent Allergy journal study reviews current knowledge regarding the generation of immune memory responses and their durability post-infection and vaccination. The researchers also provide insights into immune memory for its ability to protect against emerging VOCs.
Methods for detecting SARS-CoV-2 immune memory cells
The adaptive immune system primarily relies on the action of both T- and B-cells to elicit antigen-dependent and antigen-specific responses. After infection or vaccination, B- and T-cells that recognize a pathogen from prior exposure will respond, proliferate, and differentiate.
Thus, the cells of the adaptive immune system allow for the development of immunologic memory, comparable to the innate immune response that does not have the capacity for memory. The cells responsible for this subsequent response are memory B-cells (Bmem) and memory T-cells (Tmem).
Bmem are traditionally detected using enzyme-linked immunosorbent spot (ELISPOT), an extremely sensitive and rapid technique. Despite these advantages, ELISPOT is a time-consuming process that does not provide any information on isolated B-cells that do not recognize the antigen of interest.
Another approach that can be used to determine the antigen reactivity of Bmem or plasma cells is the immortalization of B-cell clones, which identifies the antibodies produced by a single B-cell clone. However, this method is often time-consuming and labor-intensive, limiting its applicability in certain settings.
Antigen-specific B-cells can also be identified by labeling the antigen of interest and subsequently probing the cells for their reactivity to these antigens. This approach ensures a thorough examination of the immunophenotype of these cells while also allowing the researchers to collect the cells at the end of the experiment for further analysis.
Compared to B-cell analysis, the assessment of SARS-CoV-2-specific T-cells is more challenging, as they only recognize a peptide fragment of the original antigen. Thus, researchers will use different assays to detect antigen-specific CD8+ and CD4+ T-cells.
Antigen-specific T-cells can also be assessed by stimulating peripheral blood mononuclear cells (PBMCs) with whole protein antigens to determine the different T-cells produced by this reaction, as indicated by certain activation markers. These can include intracellular cytokines such as interleukin 2 (IL-2), tumor necrosis factor α (TNF- α), and interferon γ (IFN- γ).
Antibody responses to SARS-CoV-2
Between seven to 10 days after SARS-CoV-2 infects cells, activated B-cells differentiate into plasmablasts. These plasmablasts subsequently induce the production of antibodies that typically target the spike and nucleocapsid proteins of SARS-CoV-2 by 20 days following infection.
The quantification of neutralizing antibodies (nAbs) is often used to reflect prior SARS-CoV-2 infection. After infection or vaccination, these nAbs remain stable for at least three months, with some antibody levels persisting for up to eight to 15 months after the initial antibody response.
The circulation of Bmem has also been used to assess the trajectory of COVID-19. Early in SARS-CoV-2 infection, Bmem typically expresses immunoglobulin M (IgM) and subsequently shifts towards CD21 expression.
For up to 11 months after infection, Bmem will switch to IgG at increasing levels. Additionally, CD27+ and CD71- Bmem levels may remain stable for more than 12 months following infection, thus indicating a durable B-cell memory response.
Various SARS-CoV-2-specific T-cells have been characterized, including CD8+ and CD4+ effector and memory subsets, as well as T helper cells (Tfh). Both CD4+ T-cell and Tfh cell responses remain robust for about one month following infection, whereas CD8+ T-cells remain detectable in 70-80% of convalescent samples at this time point.
These T-cell responses can remain detectable up to eight months post-infection. However, unlike Bmem, Tmem levels typically decline over time.
Immune response to vaccination
Adenoviral vector and messenger ribonucleic acid (mRNA) COVID-19 vaccines rapidly developed following the onset of the pandemic and subsequently received approval in many nations worldwide. These two types of COVID-19 vaccines were designed to produce both humoral and cellular responses against the SARS-CoV-2 spike protein.
High levels of nAbs have been detected four weeks following administration of both adenoviral and mRNA vaccines, with convalescent individuals producing much higher antibody levels as compared to naïve individuals following vaccination. Plasma cells produced following mRNA vaccination have been detected for up to seven months.
Compared to mRNA vaccines, adenoviral vaccines generate significantly lower IgG and nAbs. Antibody levels appear to peak 15-20 days following mRNA vaccination, followed by a decline in nAb levels.
SARS-CoV-2 spike-specific Bmem generated following one mRNA vaccine dose peak one month after receipt of the second vaccine dose. Interestingly, convalescent individuals generate higher Bmem levels in response to the first mRNA vaccine dose as compared to naive individuals due to pre-existing infection-induced immune memory cells. Nevertheless, infection-naïve individuals produce spike-specific Bmem that are detectable for up to six months after the second mRNA vaccine dose.
Spike-specific CD4+ and CD8+ T-cells also appear to peak within the first four weeks following completing a two-dose mRNA vaccine series. However, compared to the stable Bmem levels reported following vaccination, CD4+ and CD8+ T-cell levels appear to decline three months following vaccination.
Immunity against SARS-CoV-2 VOCs
Several SARS-CoV-2 variants of concern (VOCs) have been reported that are more infective, more likely to cause severe disease, and capable of evading vaccine-induced immunity.
To address the decreased protection conferred by vaccination against these VOCs, a third booster dose three to six months after primary vaccination has been recommended in several countries. In addition, booster vaccination has been shown to reactive Bmem, thus increasing the production of nAbs to bind to and neutralize SARS-CoV-2 VOCs.
Conclusions
The robust efforts to understand immune memory elicited after COVID-19 vaccination and/or SARS-CoV-2 infection, and the durability of these responses will assist in designing future therapies to treat vulnerable individuals. Clinical assays capable of identifying Tmem and Bmem will allow researchers to assess vaccine efficacy to determine the need and timings for subsequent booster doses.
Further research is needed to determine the capacity of immune memory in recognizing VOCs and providing protection from infection or severe COVID-19 and associated fatality. Taken together, these efforts will aid in combatting the ongoing COVID-19 pandemic.
- Hartley, G., Edwards, E., O’Hehir, R., et al. (2022). New insights into human immune memory from SARS‐CoV‐2 infection and vaccination. Allergy. doi:10.1111/all.15502.
Posted in: Medical Science News | Medical Research News | Disease/Infection News
Tags: ACE2, Allergy, Angiotensin, Angiotensin-Converting Enzyme 2, Antibodies, Antibody, Antigen, Asthma, Blood, CD4, Cell, Chronic, Cold, Coronavirus, Coronavirus Disease COVID-19, covid-19, Cytokines, Efficacy, Enzyme, Gastrointestinal Tract, Heart, Immune Response, Immune System, immunity, Immunoglobulin, Interferon, Interleukin, Intracellular, Labor, MERS-CoV, Necrosis, Obesity, Pandemic, Pathogen, Phenotype, Protein, Receptor, Research, Respiratory, Ribonucleic Acid, SARS, SARS-CoV-2, Severe Acute Respiratory, Spike Protein, Syndrome, T-Cell, Tumor, Tumor Necrosis Factor, Vaccine
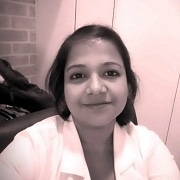
Written by
Nidhi Saha
I am a medical content writer and editor. My interests lie in public health awareness and medical communication. I have worked as a clinical dentist and as a consultant research writer in an Indian medical publishing house. It is my constant endeavor is to update knowledge on newer treatment modalities relating to various medical fields. I have also aided in proofreading and publication of manuscripts in accredited medical journals. I like to sketch, read and listen to music in my leisure time.
Source: Read Full Article